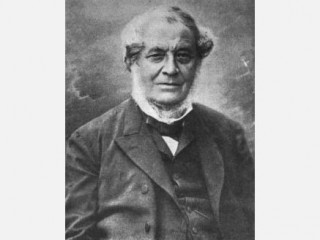
Robert Bunsen biography
Date of birth : 1811-03-31
Date of death : 1899-08-16
Birthplace : Gottingen, Germany
Nationality : German
Category : Science and Technology
Last modified : 2010-06-02
Credited as : Chemist and physicist, Bunsen battery, cesium and rubidium
19 votes so far
He demonstrated a procedure for identifying matter based on its spectral characteristics. Using his spectroscope, he discovered the elements cesium and rubidium.
Robert Bunsen was born on March 31, 1811 in Gottingen, Germany, the youngest of four sons. As his father was a professor of modern languages at the university, an academic environment would surround him from birth. After schooling in the city of Holzminden, Bunsen studied chemistry at Gottingen. He was awarded his doctorate in 1830 at the early age of 19 for a dissertation on different kinds of hygrometer. Immediately after that Bunsen set off on extensive travels, partially underwritten by the government, that took him through Germany and Paris and eventually to Vienna from 1830 to 1833.
During this trip, Bunsen visited Henschel’s machinery manufacturing plant and saw the “new small steam engine.” In Berlin, he saw the mineralogical collections of Weiss and had contact with Runge, the discoverer of aniline. Continuing on his journeys, Bunsen met with Liebig in Giessen and with Mitscherlich in Bonn for a geological trip through the Eifel mountains.
In Paris and Vienna, Bunsen visited the Sevres porcelain works and met with the outstanding chemists of the times, including famous Joseph Louis Gay-Lussac. In Paris in 1832 he also attended lectures at the famous Ecole Polytechnique. These travels allowed Bunsen the opportunity to establish a network of contacts that would stay with him throughout his illustrious career.
Upon his return to Germany, Bunsen became a lecturer at Gottingen and began his experimental studies of the insolubility of metal salts of arsenious acid. His discovery of the use of iron oxide hydrate as a precipitating agent is still the best known antidote against arsenic poisoning to this day. In two years Bunsen gained his Habilitation with work on these organometallic compounds. This was his only venture in organic/physiological chemistry.
In 1836, Bunsen was nominated to succeed Wohler at Kassel. He taught there for two years before accepting a position at the University of Marsburg. Moving from Kassel to Marsburg he took over the directorship of the Chemical Laboratory there and was appointed extraordinary professor on 7 August 1839. Bunsen has performed there his important and dangerous studies of cacodyl derivatives.
This research was his only work in pure organic chemistry and made him immediately famous within the scientific community. Cacodyl (from the Greek kakodhs – “stinking”, now named tetra-methyldiarsine) was also known as alkarsine or “Cadet’s liquid,” a product made from arsenic distilled with potassium acetate. The chemical composition of this liquid was unknown, but it and its compounds were known to be poisonous, highly flammable and had an extremely nauseating odor even in minute quantities. Bunsen’s daring experiments showed that cacodyl was an oxide of arsenic that contained a methyl radical.
These results significantly furthered the earlier work by Gay-Lussac, who had isolated the radical cyan in 1815, and that of Liebig and Wohler who published “One the radical of benzoic acid” in 1832. Typical of his research life, however, Bunsen seemed content to explore subjects of interest in his lab, but remained outside the fray that surrounded the often “violent” discussions of theoretical subjects.
Although Bunsen’s work brought him quick and wide acclaim, he nearly killed himself from arsenic poisoning and it also cost him the sight of one eye – an explosion of the compound sent a sliver of glass into his eye. Recovery was slow and painful.
His next research, still at Marburg, was on gas analysis. This seems to have begun with early (1838) work on the gases present in the blast furnaces used for making iron. Accompanied by a collaborator, Lyon Playfair, he visited England and their results were taken up by iron-masters with huge savings of fuel that would otherwise have been needlessly wasted. They suggested techniques that could recycle gases through the furnace and retrieve valuable escaping by-products such as ammonia.
From this work he went on to show how to determine the specific gravity of gases, to measure their absorption by liquids, and their rates of diffusion. Above all, he perfected the technique of eudiometry, where known volumes of gas are exploded with oxygen and the amounts of the products measured. His methods of measuring volumes of gases were described in his only publication on this subject, Gasometrische Methoden (1857). His pioneering studies of gas analysis laid the foundation of techniques still in use 100 years later.
Other work during this period concentrated on technological experiments such as the generation of galvanic currents in batteries. In 1841, instead of the expensive platinum electrode used in Grove’s battery, Bunsen made a carbon electrode. This led to large scale use of the “Bunsen battery” in the production of arc-light and in electroplating.
One of the more memorable episodes during Bunsen’s tenure at Marsburg was a geological trip to Iceland sponsored by the Danish government following the eruption of Mount Hekla in 1845. Indulging his lifelong interest in geology, Bunsen collected gases emitted from volcanic vents and performed extensive chemical analyses of volcanic rock. In addition to sampling lava gases, Bunsen investigated the theory of geyser action. Once he made daring temperature measurements of the water in the geyser tube of Iceland’s Great Geyser just before it erupted. The popular belief of his time was that the water from geysers was volcanic in origin. Bunsen took rocks from the area and boiled them in rain water.
He found that the resulting solution was quite similar to geyser water. He conducted temperature studies on the water in the geyser tube at different depths and discovered that the water was indeed hot enough to boil. Due to pressure differentials caused by the moving column of water, boiling occurs in the middle of the tube and throws the mass of water above it into the sky above. In true investigative spirit Bunsen experimented with an artificial geyser in the lab:
“To confirm his theory, Bunsen made an artificial geyser, consisting of a basin of water having a long tube extending below it. He heated the tube at the bottom andat about the middlepoint. As the water at the middle reached its boiling point, all of the phenomena of geyser action were beautifully shown, including the preliminary thundering. That was in 1846. From that day to this Bunsen’s theory of geyser action has been generally accepted by geologists.”
Heidelberg University
Bunsen and Kirchoff, a physicist trained at Konigsberg, met and became friends in 1851, when Bunsen spent a year at the University of Breslau, where Kirchoff was also teaching. Bunsen was called to the University of Heidelberg in 1852, and he soon arranged for Kirchoff to teach at Heidelberg as well.
In 1852 Bunsen succeeded Leopold Gmelin at Heidelberg. His stature was such that he attracted students and chemists from all over the world to study in his laboratory. May 1853 saw commencement of the work on the Chemical Laboratory, soon to become the largest and best-equipped lab of its kind anywhere in the world. Bunsen’s presence in Heidelberg attracted many other famous chemists of the day (August KekulE, Emil Erlenmeyer, Adolf von Baeyer, Henry Roscoe) and made the University of Heidelberg one of the major world centres of chemical research.
Bunsen ignored the current trend in organic chemistry which was fast overtaking the experimental world. Instead, Bunsen improved his earlier work on batteries: using chromic acid instead of nitric acid, he was able to produce pure metals such as chromium, magnesium, aluminum, manganese, sodium, aluminum, barium, calcium and lithium by electrolysis.
At Heidelberg, Bunsen pioneered studies in photochemsitry (with H. E. Roscoe), working with hydrogen/chlorine mixtures exposed to sunlight.
A former student of Bunsen’s believed that it was this “splendid light” from the combustion of magnesium that led Bunsen to devote considerable attention to photochemical studies. A ten year collaboration with Sir Henry Roscoe began in 1852. They took equal volumes of gaseous hydrogen and chlorine and studied the formation of HCl, which occurs in specific relationship to the amount of light received. Their results showed that the light radiated from the sun per minute was equivalent to the chemical energy of 25 x 1012 m3 of a hydrogen-chlorine mixture forming HCl.
In 1859, Bunsen suddenly discontinued his work with Roscoe, telling him: “At present Kirchhoff and I are engaged in a common work which doesn’t let us sleep… Kirchhoff has made a wonderful, entirely unexpected discovery in finding the cause of the dark lines in the solar spectrum…. thus a means has been found to determine the composition of the sun and fixed stars with the same accuracy as we determine sulfuric acid, chlorine, etc., with our chemical reagents. Substances on the earth can be determined by this method just as easily as on the sun, so that, for example, I have been able to detect lithium in twenty grams of sea water.”
Gustav Kirchhoff, a young Prussian physicist, had the brilliant insight to use a prism to separate the light into its constituent rays, instead of looking through colored glass to distinguish between similarly colored flames. Thus the fledgling science of spectroscopy, which would develop into a vital tool for chemical analysis, was born. In order to study the resultant spectra, however, a high temperature, nonluminous flame was necessary.
Robert Bunsen invented the burner that bears his name sometime in 1855. For a number of years he had been considering the problem, not only of lighting the laboratory room itself, but also developing a better heat source for lab work. Up until his invention, the flames produced had all been smokly, excessively flickering ones of low heat intensity.
The groundwork was laid three years prior when Bunsen moved to the University of Heidelberg. As a condition of his coming, he insisted on a new laboratory building and he also wanted gas piping included. The city of Heidelberg had just acquired a gas works to light the city streets. Bunsen got what he wanted.
The idea Bunsen had was simple. Instead if mixing the gas with the air right at the point of combustion, he proposed mixing the gas with the air before combustion. He went to the university mechanic, Peter Desaga, who designed and built the burner according the Bunsen’s specifications.
His son, Carl Desaga, wound up founding the firm C. Desaga, Factory for Scientific Apparatus, to handle the demands that began flowing in from all the world. Although no records exist, it is probably Peter Desaga who contributed the modern design of two large holes with a rotatable, perforated ring. Bunsen and Desaga did not apply for patent protection on their burner and it was quite soon that others began to produce their own versions. Some even went so far as to claim the invention as their own, including one person who was granted a patent on the device. Both Bunsen and Desaga were involved in writing letters to the proper authorities to refute these claims.
The Bunsen burner was the forerunner of the gas-stove burner and the gas furnace. The Bunsen burner consists of a metal tube on a base with a gas inlet at the lower end of the tube, which may have an adjusting valve; openings in the sides of the tube can be regulated by a collar to admit as much air as desired. The mixture of air and gas (optimally about 1 part gas to 3 parts air) is forced by gas pressure to the top of the tube, where it is ignited with a match.
It burns with a pale blue flame, the primary flame, seen as a small inner cone, and a secondary, almost colourless flame, seen as a larger, outer cone, which results when the remaining gas is completely oxidized by the surrounding air. The hottest part of the Bunsen flame, which is found just above the tip of the primary flame, reaches about 1,500 C (2,700 F). With too little air, the gas mixture will not burn completely and will form tiny carbon particles that are heated to glowing, making the flame luminous. With too much air, the flame may burn inside the burner tube; that is, it may strike back.
The Meker and Fisher burners, variations of the original Bunsen burner, have metallic grids to increase the turbulence of the mixture and keep the flame at the top of the tube. The Fisher burner uses forced air. There is no secondary flame dependent on surrounding air, because these improvements introduce sufficient air for complete combustion, and the heat of the primary flame is augmented.
Within five years of the development of the burner, Bunsen and Kirchhoff were deeply involved with spectroscopy, inventing yet another instrument: the Bunsen-Kirchhoff spectroscope. This vital instrument of chemical analysis can trace its ancestry to such simple components as a “prism, a cigar box, and two ends of otherwise unusable old telescopes.” From such humble beginnings came the instrument which proved to be of tremendous importance in chemical analysis and the discovery of new elements.
This spectrometer is described in the 1888 Queen Catalogue of Instruments for Physical Optics as “Bunsen’s Laboratory Spectroscope. With One prism. Consists of a collimator, with adjustable slit and a prism for comparison of spectra, a second collimator, with a photographed millimeter scale, and a telescope for examining the rays from the former two. The prism, which is of flint-glass, is inclosed in a strong metal box. All mounted upon a neat stand and packed in a strong box with lock … $55.00 [according to the 1888 price]“.
In addition to yielding a unique spectrum for each element, the spectroscope had the advantage of definite identification while only using a minimal amount of sample, on the range of nanograms to micrograms for elements like sodium and barium respectively. Using the techniques they devised, Bunsen and Kirchhoff announced the discovery of cesium (Latin caesium, “sky blue”) in the following passage: “Supported by unambiguous results of the spectral-analytical method, we believe we can state right now that there is a fourth metal in the alkali group besides potassium, sodium, and lithium, and it has a simple characteristic spectrum like lithium; a metal that shows only two lines in our apparatus: a faint blue one, almost coinciding with Srd, and another blue one a little further to the violet end of the spectrum and as strong and as clearly defined as the lithium line.”
Some of Bunsen’s enthusiasm is readily apparent in a letter to Roscoe dated November 6, 1869: “I have been very fortunate with my new metal… I shall name it cesium because of its beautiful blue spectral line. Next Sunday I expect to find time to make the first determination of its atomic weight.”
In 1861, only a few months following their cesium discovery, Bunsen and Kirchhoff announced the discovery of yet another new alkali metal. Two hitherto undiscovered violet spectral lines in an alkali of the mineral lepidolite were attributed to a new element, rubidium (Latin rubidus, “darkest red colour”). Bunsen and Kirchhoff’s combined genius quickly paved the way for others to claim elemental discoveries.
The spectroscope served as a springboard by which five new elements were discovered. These included thallium (Crookes, 1861), indium (Reich and Richter, 1863), gallium (Lecoq de Boisbaudran, 1875), scandium (Nilson, 1879) and germanium (Winkler, 1886). Fittingly, Bunsen’s original vision of analyzing the composition of the stars was realized in 1868 when helium was discovered in the solar spectrum.
In 1870 Bunsen devised a sensitive ice calorimeter that measured the volume rather than the mass of the ice melted. This allowed him to measure the metals’ specific heat to find their true atomic weights. The ice calorimeter of Bunsen finds the number of melted grams of ice by measuring volumes. 1 g of ice occupies 1.0908 cm3, 1 g of water 1.0001 cm3. When 1 g of ice melts it reduces its volume by 0.0907 cm3. The measured reduction in volume of melting ice indicates the number of grams which have melted. The figure (left) shows the instrument, completely blown out of glass.
The U-tube C, the wider part g of which ends above in a small test tube for the body to be examined, contains above b water and ice, mercury from b into the calibrated capillary S . The instrument has protection against external heat effects by being surrounded by a mixture of ice and water. You place the body, heated to to, into the tube filled with water at temperature 0o.
The body passes its heat to the water and through it to the ice; the ice melts, the volume occupied by ice and water reduces and allows the mercury to rise above b. The displacement of the mercury column in S indicates the number of cm3, which have risen above b. 11.03 g of melted ice correspond to a volume change of 1 cm3. The Bunsen calorimeter allows to make very exact measurements and requires only a few grams of the substance to be investigated, but demands strict conformity with several intricate rules (for example, for the production of the ice mantle).
During this period, he also pressed magnesium into wire; the element came into general use as an outstanding illuminating agent.
Bunsen also invented the filter pump (1868), the vapour calorimeter (1887) and grease-spot photometer used to compare intensity of similar light sources (1844), a new valve, Bunsen-effuser (determines gas density by velocity measurement of gas drain through a small hole).
Throughout his professional life, Bunsen’s personal life centered around his laboratory and his students. Never marrying, Bunsen often took on the introductory courses that were shunned by other colleagues. During the one hundred hours of lectures presented each semester, Bunsen emphasized experimentation and tabulated summaries and patiently introduced students to the world of analytical chemistry.
Bunsen’s habit was to assign a scientific task to his students and then to work with a student only as long as required to reach some measure of independence. Many principal players in the history of chemistry can trace their chemical roots back to Bunsen’s laboratory. Two of his more famous students were Dmitri Mendeleev and Lothar Meyer.
According to accounts, Bunsen was one of the more modest of giants: “He never said: ‘I have discovered,’ or ‘I found’… He was characterized by extraordinary, distinguished modesty. That does not mean that he was not conscious of his own value. He knew how to use it at the right time and in the right company; he even had a considerable degree of very sound egotism.”
The scientific world held Bunsen in high esteem for much of his long professional life. In 1842 he was elected to the Chemical Society of London and the Academie des Sciences in 1853. He was named a foreign fellow of the Royal Society of London in 1858, receiving its Copley Medal in 1860. Bunsen and Kirchhoff were recipients of the first Davy Medal in 1877. The Albert Medal was awarded in 1898 in recognition of Bunsen’s many scientific contributions to industry. Of these honors, Bunsen once remarked, “Such things had value for me only because they pleased my mother; she is now dead.”
Upon his retirement at the age of 78, Bunsen left the chemical work behind, returned to his first love of geology, keeping up with the latest developments in the field and corresponding with his old friends such as Roscoe, Kirchhoff and Helmholtz.
Bunsen died August 16, 1899 after a peaceful three day sleep, leaving behind a glowing legacy of discoveries and technological advances that allowed the world of chemistry to burn brightly. Nine years after his death (1908) a monument was erected in his honour (Hauptstrasse).