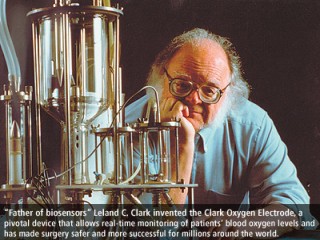
Leland C. Clark biography
Date of birth : 1918-12-04
Date of death : 2005-09-25
Birthplace : Rochester, New York, U.S.
Nationality : American
Category : Science and Technology
Last modified : 2010-05-31
Credited as : Biochemist, Inventor of Oxycyte, Clark Oxygen Electrode
3 votes so far
Leland C. Clark was born in 1918. When Leland Clark started high school and discovered that science was an academic discipline, complete with course work, lab sessions and grades, he said, “It was like discovering that you could get a grade for eating chocolate ice cream.” One of the few students to score a perfect 100 on the New York State Regents science exam, Dr. Clark attended Antioch College and the University of Rochester School of Medicine, where he received his Ph.D. in biochemistry and physiology. Later, he taught at Antioch, the University of Cincinnati and the University of Alabama.
It is generally agreed that biosensor history started in 1962 and that the progenitor of the biosensor was the American scientist Leland C. Clark. Clark had studied the electrochemistry of oxygen gas reduction at platinum (Pt) metal electrodes, pioneering the use of the later as an oxygen- (and therefore chemi-) sensor. In fact, Pt electrodes used to detect oxygen electrochemically are often referred to generically as “Clark electrodes”. More than almost any single invention, the Clark Oxygen Electrode has revolutionized the field of medicine for the past 50 years. The Clark oxygen electrode, which he invented in 1954, remains the standard for measuring dissolved oxygen in biomedical, environmental, and industrial applications. The electrode quickly measures blood oxygen levels, enabling doctors to perform 750,000 open-heart surgeries each year. Oxygen monitoring is now a requirement for hospital accreditation. It is also used to measure oxygen levels in rivers and oceans to protect wildlife populations.
The electrochemical reduction of oxygen was discovered by Heinrich Danneel and Walter Nernst in 1897. Polarography using dropping mercury was discovered accidentally by Jaroslav Heyrovsky in Prague in 1922. This method produced the first measured oxygen concentration values in plasma and blood in the 1940s. Brink, Davies, and Bronk implanted platinum electrodes in tissue to study oxygen supply, or availability, from about 1940, but these bare electrodes became poisoned when immersed in blood. Leland Clark sealed a platinum cathode in glass and covered it first with cellophane; he then tested silastic and polyethylene membranes. In 1954 Clark conceived and constructed the first membrane-covered oxygen electrode having both the anode and cathode behind a nonconductive polyethylene membrane. The limited permeability of polyethylene to oxygen reduced depletion of oxygen from the sample, making possible quantitative measurements of oxygen concentration in blood, solutions, or gases. This invention led to the introduction of modern blood gas apparatus.
Clark oxygen electrodes have a thin organic membrane covering a layer of electrolyte and two metallic electrodes. Oxygen diffuses through the membrane and is electrochemically reduced at the cathode. There is a carefully fixed voltage between the cathode and an anode so that only oxygen is reduced. The greater the oxygen partial pressure, the more oxygen diffuses through the membrane in a given time. This results in a current that is proportional to the oxygen in the sample. Temperature sensors built into the probe on some advanced measurement systems allow compensation for the membrane and sample temperatures, which affect diffusion speed and solubility. The meter uses cathode current, sample temperature, membrane temperature, barometric pressure and salinity information to calculate the dissolved oxygen content of the sample in either concentration (ppm) or percent saturation t% Sat). The voltage for the reduction can either be supplied electronically by the meter (potentiometric oxygen electrode) or dissimilar metals may be used for the two electrodes, picked so that the correct voltage is generated between them (galvanic electrode).
This is a polarographic electrode used for measuring the concentration of oxygen in liquid medium (e.g. blood) and gases. The sample is brought into contact with a membrane (usually polypropylene or Teflon) through which oxygen diffuses into a measurement chamber containing potassium chloride solution. In the chamber are two electrodes; one is a reference silver/silver chloride electrode and the other is a platinum electrode coated with glass to expose only a tiny area of platinum (e.g. 20 mm diameter).
The electric current flow between the two electrodes when polarized with a potential of -600 mV (vs. Ag/AgCl) determines the oxygen concentration in the solution. Originally developed for measuring oxygen gas, it is only a matter of polarity, whether the electrode senses hydrogen or oxygen gas. For hydrogen measurements +600 mV (vs. Ag/AgCl) are supplied.
The reaction is very sensitive to temperature and to maintain a linear relationship between the oxygen (or hydrogen) concentration and the current measured the electrode temperature must be controlled within 0.1 oC. The electrode is calibrated using two gas mixtures of known oxygen (or hydrogen) concentration. Such oxygen sensitive electrodes are used in the blood gas analyser in the clinical chemistry laboratory or in intensive care areas.
The Clark-type electrode consists of a Pt- (A) and a reference Ag/AgCl-electrode (B) covered by a film of half-saturated KCl electrolyte (C) enclosed within a Teflon membrane (D) which is held in place by a rubber ring (E). The voltage supply (F) and the electronic instrument for the measurements of the current output is shown (G).
Clark had the ingenious idea of placing very close to the surface of the platinum electrode (by trapping it physically against the electrode with a piece of dialysis membrane) an enzyme that reacted with oxygen. He reasoned that he could follow the activity of the enzyme by following the changes in the oxygen concentration around it, thus a chemosensor became a biosensor. Based on this experience and addressing his desire to expand the range of analytes that could be measured in the body, he made a landmark address in 1962 at a New York Academy of Sciences symposium in which he described how “to make electrochemical sensors (pH, polarographic, potentiometric or conductometric) more intelligent” by adding “enzyme transducers as membrane enclosed sandwiches”.
The concept was illustrated by an experiment in which glucose oxidase was entrapped at a Clark oxygen electrode using dialysis membrane. The decrease in measured oxygen concentration was proportional to glucose concentration. In the published paper (Clark, L.C. Jnr. Ann. NY Acad. Sci. 102, 29-45, 1962), Clark and Lyons coined the term enzyme electrode. Clark’s ideas became commercial reality in 1975 with the successful re-launch (first launch 1973) of the Yellow Springs Instrument Company (Ohio) glucose analyser based on the amperometric detection of hydrogen peroxide. This was the first of many biosensor-based laboratory analysers to be built by companies around the world.
In addition to the Clark Oxygen Electrode, he developed the first heart-lung machine that could be completely disassembled and sterilized, as well as the technology behind the glucose biosensor used by millions of diabetics and other patients every day.
For more than 30 years, Dr. Clark has pursued his dream of creating artificial blood. While making notable progress toward a solution, that technology remains in the very early stages of development.
The first real success in fluid breathing came in 1966, with Dr. Leland Clark’s “liquid-breathing-mouse” experiment. Dr. Clark realized that oxygen and carbon dioxide were very soluble in fluorocarbon liquids (like freon). Assuming that the alveoli of the lungs should be capable of drawing oxygen out of the fluid and replacing it with carbon dioxide, Clark figured that these fluorocarbons should support respiration of animals. Performing the first tests on anesthetized mice, Dr. Clark temporarily paralyzed each animal and placed a tube down the trachea, inflating a cuff inside the airway to provide a seal and ensure that no air entered the lungs, and no solution leaked out.
After bubbling oxygen through the fluorocarbon, the oxygenated fluid was pumped into the animals’ lungs, and recirculated (about 6 cycles of inhalation and exhalation per minute). Most of the animals who were kept in the fluid for up to an hour survived for several weeks after their removal, before eventually succumbing to pulmonary damage. Autopsies uniformly revealed that the lungs appeared congested when collapsed but normal when inflated. Some of the early problems Clark encountered seemed to be due to the size of the animals’ airway. The tiny size physically limited the amount of fluid that could get into the lungs. For that and other reasons, carbon dioxide tended to build up in the system: it simply couldn’t be removed fast enough.
Dr. Leland Clark of Cincinnati invented a blood substitute allowing this living mouse to breathe in the liquid, while goldfish inhabit the water floating on top.
Dr. Clark discovered that the length of time the mice could survive in the fluid was directly related to the fluorocarbon’s temperature: the colder the fluid, the lower the respiration rate which in turn prevented carbon dioxide buildup. The only way around the problem, therefore, was to induce hypothermia in the animals. This technique seemed to give him the most success, as one animal survived over 20 hours breathing fluid at 18 degrees Centigrade.
All animals in the earliest studies suffered pulmonary damage, but whether that was due to toxic impurities of the fluorocarbon, chemical interaction of the fluorocarbon with the lung, or some unknown effect was undetermined. This mystery of the pulmonary damage, as well as the problem of carbon dioxide elimination and the fact that the fluorocarbon tended to be retained by the body tissues, would have to be solved before the process could be attempted on human subjects.
Dr. Clark, one of the century’s most prolific biomedical inventors and researchers, is recognized for pioneering several medical milestones credited with saving thousands of lives and advancing the technology of modern medicine. His research accomplishments include the development of the first successful heart-lung machine, the advancement of technology leading to the development of one of the first intensive care units in the world, and pioneering research in biomedical applications of perfluorocarbons and biosensors. He has published more than 400 scientific papers in biomedicine and has generated numerous US and foreign patents, mainly in the field of medical instrumentation and fluorocarbons.
He is the recipient of numerous honors and awards including induction into the National Academy of Engineering and the Engineering and Science Hall of Fame.
In 1985, Leland Clark received the American Physiological Society’s Heyrovsky Award, in recognition of the invention of the membrane polarographic oxygen electrode. He is a member of NAE, National Association for Biomedical Research, and the American Association for the Advancement of Science.