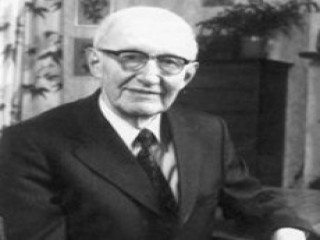
Ernest Walton biography
Date of birth : 1903-10-06
Date of death : 1995-06-25
Birthplace : Dungarven, County Waterford, Ireland
Nationality : Irish
Category : Science and Technology
Last modified : 2011-04-02
Credited as : Nuclear physicist, Nobel Prize in Physics, disintegration of an atomic nucleus
Physicist Ernest Walton shared the achievement of the first artificial disintegration of an atomic nucleus without the use of radioactive elements. For this work, Walton and John D. Cockroft shared the 1951 Nobel Prize in physics.
Ernest Walton was an Irish experimental physicist who gained renown for achieving, with physicist John D. Cockcroft, the first artificial disintegration of an atomic nucleus, without the use of radioactive elements. Their breakthrough was accomplished by artificially accelerating a beam of protons (basic particles of the nuclei of atoms that carry a positive charge of electricity) and aiming it at a target of lithium, one of the lightest known metals. The resultant emission of alpha particles, that is, positively charged particles given off by certain radioactive substances, indicated not only that some protons had succeeded in penetrating the nuclei of the lithium atoms but also that they had somehow combined with the lithium atoms and had been transformed into something new. Although the process was not an efficient energy producer, the work of Walton and Cockcroft stimulated many theoretical and practical developments and influenced the whole course of nuclear physics. For their pioneering work, Walton and Cockcroft shared the 1951 Nobel Prize in Physics.
Ernest Thomas Sinton Walton was born October 6, 1903, in Dungarven, County Waterford, in the Irish Republic. His father, John Arthur Walton, was a Methodist minister, while his mother, Anna Elizabeth (Sinton) Walton, was from a very old Ulster family, who had lived in the same house in Armagh for over two hundred years. The young Walton was sent to school at Belfast's Methodist College, where he demonstrated an aptitude for science and math. It was no surprise, then, that he decided to enroll in math and experimental science at Dublin's Trinity College in 1922. He graduated in 1926 with a B.A. degree, in 1928 with a M.Sc., and in 1934 with a M.A.
The following year he headed to Cambridge University, England, on a Clerk Maxwell research scholarship. There, he joined the world-famous Cavendish Laboratory, headed by the great New Zealand-born physicist Ernest Rutherford. Walton was assigned cramped laboratory space in a basement room. While his quarters were less than luxurious, he was at least blessed by having roommates with whom he struck up an immediate friendship, physicists T. E. Allibone and John D. Cockcroft. Walton would go on to make scientific history, in collaboration with the latter, for a project that would pave the way for the development of the atom bomb. At the suggestion of Rutherford, Walton began attempting to increase the velocity of electrons (the negatively charged particles of the atom) by spinning them in the electric field produced by a changing circular magnetic field as a method of nuclear disintegration. Although the method was not successful, he was able to figure out the stability of the orbits of the revolving electrons, and the design and engineering problems of creating an accelerating machine with minimal tools and materials. This early work of Walton's later led to the development of the betatron, that is, a particle accelerator in which electrons are propelled by the inductive action of a rapidly varying magnetic field.
Next, Walton tried to build a high frequency linear accelerator. His goal was to produce a stream of alpha particles traveling at high speed which could be used to shed light on various aspects of the atomic nucleus. Rutherford had long been keen to get his hands on such a source of alpha particles but despaired of any short-term breakthrough. As Walton's work progressed, Rutherford's wish was granted sooner than he expected.
What was needed was a fundamentally different way of viewing the problem. Walton and his colleagues at the Cavendish were trying to accelerate electrons to a speed sufficient to enable them to penetrate an atomic nucleus. Such high velocities were necessary, they believed, in order to counteract the repulsive charge of the nuclei. The speeding electrons, they figured, would literally bully their way through. However, achieving such high speeds was easier said than done. It required the application of enormous amounts of electricity, about four million volts, which at that time was impossible to generate in a discharge tube (a tube that contains a gas or metal vapor which conducts an electric discharge in the form of light). A crucial breakthrough came in 1929, when the Russian physicist George Gamow visited the Cavendish laboratory. With physicist Niels Bohr in Copenhagen, he had worked out a wave-mechanical theory of the penetration of particles, in which they believed particles tunneled through rather than over potential barriers. This meant that particles propelled by about 500,000 volts, as opposed to millions, could possibly permeate the barrier and enter the nucleus if present in sufficiently large numbers. That is, one would need a beam of many thousands of millions of moving particles to produce atomic disintegrations that would be capable of being observed.
Rutherford gave Walton and Cockcroft the go-ahead to test the supposition. It was a measure of his confidence in them—the high voltage apparatus they constructed to enable them to accelerate atomic particles cost almost £1,000 (British pounds) to build. It was an enormous sum in those days, and represented almost the entire annual budget for the laboratory.
The machine, the first of its kind ever built, and today on view at the London Science Museum in South Kensington, was built out of an ordinary transformer, enhanced by two stacks of large condensers (or what would today be called capacitors), which could be turned on and off by means of an electronic switch. This arrangement generated up to half a million volts, which were directed at an electrical discharge tube. At the top of the tube protons were produced. The velocity of the protons was increased into a beam which could be used to hit any mark at the bottom of the tubes. Although it would be considered primitive by today's standards, their apparatus was, in fact, an ingenious construction, cobbled together from glass cylinders taken from old fashioned petrol pumps, flat metal sheets, plasticene, and vacuum pumps. The current generated by the discharge tube was almost one hundred-thousandth of an ampere, which meant that about 50 million protons per second were being produced. The availability of such a large and tightly controlled source of particles— compared with that produced by, say, a radioactive source—greatly increased the odds of a nucleus being penetrated by the speeding atomic particles.
Halfway through 1931, while their experiment was still in its early stages, Walton and Cockcroft were forced to vacate their subterranean basement when it was taken over by physical chemists. They were obliged to deconstruct their installation and build it again. As it happened, it turned out to be a lucky break. Their new laboratory was an old lecture theater, whose high ceiling was much more suitable for their purposes. When Walton and Cockcroft went to reassemble their massive apparatus, they used the opportunity to introduce a few modifications. This time around, they incorporated a new voltage multiplying circuit, which Cockcroft had just developed, into their apparatus. It took them until the end of 1931 just to produce a steady stream of five or six hundred volts.
When the accelerator was finally completed, they restarted the laborious process of trying to penetrate an atomic nucleus using a stream of speeded up protons. They positioned a thin lithium target obliquely across from the beam of protons in order to observe the alpha particles on either side of it. In order to detect the alpha particles that they hoped would be produced, they set up a tiny screen made of zinc sulfide, which they observed with a low-power microscope, a technique borrowed from Rutherford.
The first few months of 1932 were spent in rendering the installation more reliable and measuring the range and speed of the accelerated protons. It was not until April 13, 1932, that they achieved a breakthrough. On that fateful date, Walton first realized that their experiment had been successful. On the tiny screen, he detected flashes, called scintillations. These indicated that not only had the steam of protons succeeded in boring through the atomic nuclei but also that, in the process, a transformation had occurred. The speeding protons had combined with the lithium target to produce a new substance, the alpha particles, which appeared on the screen as scintillations.
Walton and Cockcroft confirmed these observations using a paper recorder with two pens, each operated by a key. Walton worked one key, Cockcroft the other. When either noticed a flash, he pressed his key. As both keys were consistently pressed at the same time, it was clear that the alphas were being emitted in pairs. The implication was that the lithium nucleus, with a mass of seven and a charge of three had, on contact with an accelerated proton, split into two alpha particles, each of mass four and charge two. In the transformation, a small amount of energy was lost, equivalent to about a quarter of a percent of the mass of lithium.
Walton and Cockcroft's achievement was ground-breaking and historic in many ways. It represented the first time that anyone had produced a change in an atomic nucleus by means totally under human control. They had also discovered a new energy source. Furthermore, they had confirmed George Gamow's theory that particles could tunnel or burrow their way into a nucleus, despite the repulsion of the electrical charges. And finally, they furnished a valuable confirmation of physicist Albert Einstein's theory that energy and mass are interchangeable. The extra energy of the alpha particles, when allowance was made for the energy of the proton, exactly corresponded to the loss of mass.
Walton and Cockcroft's achievement was announced in a letter in Nature and later at a meeting of the Royal Society of London on June 15, 1932. By that time, they had succeeded in splitting the nuclei of 15 elements, including beryllium, the lightest, to uranium, the heaviest. All produced alpha particles, although the most spectacular results were obtained from fluorine, lithium, and boron. The news caused a sensation throughout the world. As a result of their discovery, Walton and Cockcroft were the star attractions at the Solvay Conference, an important gathering of international physicists, held in 1933, and at the International Physics Conference, held in London in 1934.
Walton and Cockcroft's particle accelerator spawned many more sophisticated models, including one built by their colleague physicist Marcus Oliphant at the Cavendish. It was capable of producing a more abundant supply of particles; not only protons, but also deuterons (nuclei of heavy hydrogen). With this, many groundbreaking nuclear transformations were carried out. Their invention also inspired the American nuclear physicist Ernest Orlando Lawrence to build a cyclotron, a cyclical accelerator, capable of reaching tremendous speeds. Although scientists in the close of the twentieth century may regard the equipment Walton and Cockcroft used as primitive, the basic idea behind the particle accelerator has stayed the same.
In 1932, Walton received his Ph.D from Cambridge and two years later returned to Dublin as a fellow of Trinity College, his reputation preceding him. That same year he married Winifreda Wilson, a former pupil of the Methodist College, Belfast. They had two sons and two daughters, Alan, Marian, Philip, and Jean.
The next few years passed rather uneventfully for Walton. While his erstwhile partner, John D. Cockcroft went from one high profile position to another, Walton preferred to remain slightly aloof from the mainstream of physics. He concentrated instead on establishing his department's reputation for excellence. His efforts were rewarded in 1946 when he was appointed Erasmus Smith Professor of Natural and Experimental Philosophy.
In 1951, almost twenty years after achieving the breakthrough that changed the face of nuclear physics, Walton and Cockcroft finally achieved the recognition that many believed was long overdue. The Nobel Prize for Physics was awarded to them jointly for their pioneering work on the transmutation of atomic nuclei by artificially accelerated atomic particles. The following year, Walton became chairman of the School of Cosmic Physics of the Dublin Institute for Advanced Studies. He was elected a senior fellow of Trinity College in 1960.
Outside of his scientific work, Ernest Walton was active in committees concerned with the government, the church, research and standards, scientific academies, and the Royal City of Dublin Hospital. He died on June 25, 1995, at the age of 91.