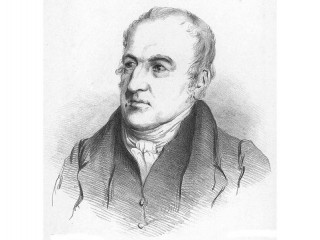
Barlow Peter biography
Date of birth : 1776-10-13
Date of death : 1862-03-01
Birthplace : Norwich, England
Nationality : British
Category : Science and Technology
Last modified : 2010-06-02
Credited as : Mathematician and physicist, Barlow lens Barlow's Tables, The Ladies' Diary
0 votes so far
In 1801, Barlow was appointed assistant mathematics master at the Royal Military Academy, Woolwich, and retained this post until 1847. He contributed articles on mathematics to The Ladies' Diary as well as publishing books such as:
- An Elementary Investigation of the Theory of Numbers (1811)
- A New Mathematical and Philosophical Dictionary (1814)
- New Mathematical Tables (1814).
The latter became known as Barlow's Tables and gives squares, cubes, square roots, cube roots, and reciprocals of all numbers from 1 to 10,000. These tables were regularly reprinted until 1965, when computers rendered them obsolete. Barlow also contributed largely to the Encyclopaedia Metropolitana.
He invented two varieties of achromatic (non-colour-distorting) telescope lenses known as Barlow lenses. In 1819, Barlow began work on the problem of deviation in ship compasses caused by the presence of iron in the hull.
For his method of correcting the deviation by juxtaposing the compass with a suitably shaped piece of iron, he was awarded the Copley Medal. He also designed in 1822 one of the first models of an electric motor supplied by continuous current.
Peter Barlow was born at Norwich on October 13, 1776. The events during his first years of his life are quite unknown. It is known that he kept a school and gained some scientific knowledge.
He became a correspondent to the ‘Ladies’ Diary’, then under the management of a Dr. Hutton, professor of mathematics at Woolwich. By his advice Barlow sought and obtained the post of assistant mathematical master in 1801. He was promoted to the post of professor of mathematics in the Royal Military Academy, Woolwich. His position brought him access to the arsenal, foundry and dockyard for his experiments and allowed Barlow contact with colleagues with similar interests. Barlow was one of a group including Edward Sabine, Charles Babbage, John Herschel, and Michael Faraday interested in magnetic and geomagnetic subjects.
He became sufficiently well established as a leading authority on mathematics that after a while he was asked to contribute various articles on mathematics for encyclopaedias. In addition to these articles, Barlow also published several important books, for example in 1811 he published “An Elementary Investigation of the Theory of Numbers” and three years later he published “A New Mathematical and Philosophical Dictionary”.
In 1814 he published his the most known book entitled “New Mathematical Tables”. These soon became known as Barlow’s Tables and this work gives factors, squares, cubes, square roots, reciprocals and hyperbolic logarithms of all numbers from 1 to 10 000.
This book: “… was considered so accurate and so useful that it has been regularly reprinted ever since.” In the mathematical library at the University of St Andrews there are several well worn copies of these tables which must have been used intensely for many years. Today, however, they are only of historical interest since they were made completely obsolete by calculators and computers.
Peter Barlow painted by Samuel Cousins, National Portrait Gallery, London
In 1817 his then most useful book ‘Essay on the Strength of Timber and other Materials’ gave important information to engineers. These values were gained through numerous experiments in the dockyard of Woolwich.
Barlow’s next major contribution makes his name still well known by amateur astronomers today. He invented the Barlow lens, a telescope lens consisting of a colourless liquid between two pieces of glass. The “Barlow lens”, a modification of this telescope lens, is a negative achromatic combination of flint glass and crown glass. It is a negative lens used to extend the effective focal length of a telescope objective.
His optical experiments began about 1827. There were several experiments to correct a single lens for chromatic aberration with concave lenses. These correctors were first placed near the first lens, but some opticians moved the concave lens further down the tube. This arrangement was described 1828 by Rogers in a paper to the Astronomical Society. By this a 3 inch concave flint lens was sufficient to correct a 9 inch crown glass. Smaller lenses near the focus would do the colour correction, but have to have steeper curves which would introduce spherical aberration.
The first scope with this arrangement of lenses was made by G. Dollond for Barlow. Making own experiments on achromatic lenses Barlow had some difficulty in getting flint-glass. He replaced the flint by the liquid carbon-disulphide which he contained in glass. This ‘liquid lens’ was only half the diameter of the front lens and placed in the middle of the telescope tube between the front lens and the eyepiece.
The first two telescopes he build with this element had 3 and 6 inches aperture and were corrected for colour and curvature by a concave-convex glass container filled with the liquid. Supported by a grant from the Board of Longitude he build a 7.8 inch refractor, and was willing to try to build a 24 inch telescope.
The Royal Society in 1831 appointed a committee to report on the practicability of this design in this size. The committee requested a 8 inch telescope with 105 inch focal length for further testing. This last telescope of this design was made in 1832 by Dollond. Herschel, Airy and Smyth examined it. They found the light gathering good but rated the chromatic and spherical aberration not enough corrected. The 24 inch was never build.
The now famous ‘Barlow Lens’ is the result of a collaboration of Barlow with George Dollond. Barlow calculated a concave achromatic lens which Dollond made in 1833 and mounted to a telescope. Dawes employed it first while measuring close double stars. He and Smyth commented favourably on this construction. The invention of this new optical element was presented to the Royal Society by Dollond.
He was admitted to the Astronomical Society and sat on the committee for the improvement of the ‘Nautical Almanac’ in 1829-30. Beside the above mentioned books Barlow contributed several articles for the ‘Encyclopaedia Metropolitana’ and Rees’s ‘Encyclopaedia’. The Royal Society’s ‘Catalogue of Scientific Papers’ lists 49 contributions by Barlow to scientific periodicals.
Barlow invented the technique of fixing a small piece of iron close to a ships compass to compensate for the large deviations due to the increasing quantities of iron in ship construction. After tests in various latitudes it was shown that this did not work on ships build wholly of iron. However, for this invention he received a grant of 500 pounds from the Board of Longitude, from emperor Alexander in Russia a golden watch and chain, and in 1821 he was being elected to the Royal Society and receiving its gold Copley medal for his work on magnetism. Between 1823 and 1833 much of his work was on the field of magnetism and electro-magnetism. He even made experiments in signalling by electricity. In a letter he described experiments on the influence of iron on the rates of chronometers.
Barlow’s study of terrestrial magnetism was also a fundamental study. Barlow’s first published mention of magnetism was in the “Mathematical and Philosophical Dictionary” of 1814. Believing the earth to act as a giant magnet he wrote: “Magnetism of the Earth, is that property of the terrestrial globe, from which the magnetism of the ordinary magnets, the direction of the magnetic needle, and other phenomena are derived; and upon which they necessarily depend. This is obvious, since almost all the phenomena, which may be exhibited with a usual magnet, may be also exhibited with the earth…”
In 1819 Barlow’s first magnetic experiments were reported in the ‘Edinburgh Philosophical Journal’. His investigations focused on deflections of the compass needle produced by ship-board iron. These deviations caused increasing navigational problems as larger amounts of iron were used to outfit and construct nineteenth-century ships. Using a compass and a large iron sphere, Barlow determined a “plane of no attraction” or set of points where the needle behaved as if no iron were present. He then systematically moved the compass in circles around the iron ball, attempting to derive a general law for the needle’s deflections from the plane of no attraction. The law deduced from these experiments allowed Barlow to compute the deviation for any compass position around the sphere. Also the needle deflected the same amount whether the sphere was hollow or solid. Barlow concluded that magnetic power resided only in or near the surface of the objects.
Extending and refining his experiments, Barlow summarized the results in “An Essay on Magnetic Attractions” in 1820. He found that a properly-placed iron plate near the ship’s compass could compensate for compass deflections caused by ship-board iron. This correction allowed for more accurate and safer navigation. The bulk of the work dealt with the application of this corrective plate. At the end of the essay, Barlow addressed theoretical concerns:
“At present I have hinted at no hypothesis explanatory of the law of action… between the iron and compass. We know that, agreeably to the theory, first, I believe, advanced by Gilbert, but since adopted and extended by Coulomb, Biot, and others, the ball of iron which I have employed in my experiments being placed in the neighborhood of the great terrestrial magnet, has itself acquired a certain portion of magnetic influence; its upper part possessing the boreal, and the lower, the austral quality.”
Barlow found that the complicated analysis resulting from such a theory rendered “it wholly useless as a practical theory.”
In 1804 Jean-Baptiste Biot had mathematically demonstrated that, if the earth acted like a lodestone, then the poles must be indefinitely near each other at the center of the earth to fit with the observations. Barlow commented on Biot’s finding:
“if this computation [Biot's] prove any thing, it is, that the hypothesis of the earth, containing within itself two magnetic poles, is altogether erroneous; for what idea can we have of an infinitely small magnet… giving directions to bodies at the distance of 4000 miles?”
Given this difficulty and others, Barlow concluded that the earth was not a giant magnet. Research over the next few years strengthened this rejection.
Explaining magnetism in terms of imponderable fluids was not a new idea in the nineteenth century. In the 1780s Charles Coulomb had elaborated a two fluid theory of magnetism paralleling his earlier theory of electricity. Barlow, accepting some version of a subtle fluid theory, did not specify which one until the second edition of “An Essay on Magnetic Attractions” in 1823. This expanded version was divided into three parts. Part one extended the experiments related to the corrective plate.
In the second part, he developed his theory of magnetism. Barlow noted that soon after the publication of his 1820 Essay, Charles Bonnycastle undertook to deduce the several laws arising out of the [Barlow's] experiments, from a theory, exceedingly simple in itself, founded on a supposed similarity of action between electrified and magnetized bodies, and employing accordingly the principles laid down by [Simeon-Denis] Poisson in… 1811, for establishing the laws of action in the former class of bodies.
Barlow modified Bonnycastle’s version of Poisson’s two fluid theory of electricity. With this theory in mind, Barlow derived formulae for predicting the deflections of a magnetic needle at any position from an iron sphere. Then he compared the computed results with his colleague, Samuel Hunter Christie’s, observations. From this comparison Barlow concluded that “it would be useless to expect a closer approximation between theory and practice.”
Next, Barlow addressed terrestrial magnetism. He considered the earlier formulae when the variable designating the earth’s magnetic intensity went to zero. The iron sphere, he wrote, “will, in miniature, resemble the action of the terrestrial globe, and the laws which we thus deduce ought to be analogous to those obtained from observations in different parts of the earth…”
The computations closely coincided with known observations. Barlow commented, “Hitherto we have found a very close approximation between the laws of magnetism appertaining to a simple iron ball and the observed magnetic phenomena of the earth.” However, when he applied the analogy to predict the position of the terrestrial magnetic axis, it failed to yield a close match.
Despite the discrepancies, he insisted that his hypothesis was consistent with known principles. Barlow also assumed that the earth induced magnetism in iron objects. This was reflected in the title of part two, “A Theoretical Investigation of the Laws of Induced and Terrestrial Magnetism.” But if the earth induced magnetism to iron bodies then what induced magnetism in the earth itself? In the next several years Barlow addressed this question.
In 1820 the study of terrestrial magnetism received impetus from Hans Christian Oersted’s discovery that electric current in a wire deflected a magnetic needle. In 1822, Thomas Seebeck found that differentially heating a circuit of appropriate metals produced an electric current. Electromagnetism and thermoelectricity gave those who believed in the “cosmical” view renewed hope for a synthesis of “telluric” forces.
Humboldt linked variations in the three magnetic components with the motions of thermal, chemical and luminous aspects of electromagnetism. Charles Babbage and John Herschel proposed that atmospheric electricity arose from the thermoelectric interaction of sky and earth, thus producing terrestrial magnetism by induction. In the 1820s, André-Marie Ampere proposed that electric fluids were the cause of magnetism. Barlow followed this trend in unifying natural forces.
In part three of his 1823 Essay, Barlow had reported on his repetition of the experiments of Oersted, Ampere, Faraday, and others. He had explained electromagnetism in terms of two galvanic and two magnetic fluids without speculating on terrestrial magnetism.
However, in 1825 Barlow wrote in a letter to John Herschel, “there are strong reasons for assuming, that the magnetism of the earth is of that kind which we call induced magnetism; but at present we have no knowledge of the inductive principle.” That same year Poisson had remarked that the question of whether magnetic fluids were distinct or mere modifications of electricity was still uncertain. By 1831 Barlow decided this question for himself in support of Ampere’s idea. This was evident in his paper titled, “On the probable Electric Origin of all the Phenomena of Terrestrial Magnetism.”
In this paper Barlow observed that the earth’s magnetic intensity remains relatively constant, yet the earth’s position is constantly changing. Hence, terrestrial magnetism could not be induced by an outside body; the cause must be internal. He wished to show that “all terrestrial magnetic phenomena are due only to electricity, and that magnetism, as a distinct quality, has no real existence.” Barlow said that an artificial globe with galvanic currents distributed across its surface would “exhibit, while under electrical induction, all the magnetic phenomena of the earth.
” A wooden globe with latitudinal grooves cut in it was constructed to test this hypothesis. In these grooves nearly ninety feet of copper wire were laid. After refining his model Barlow concluded: “Nothing can be expected nor desired to represent more exactly on so small a scale all the phenomena of terrestrial magnetism, than does this artificial globe…”
This electrified globe demonstrated a force “competent to produce all the phenomena of terrestrial magnetism, without the aid of any body usually called magnetic” and showed that magnetism has “no real existence in nature.” Barlow further speculated by commenting on thermoelectricity: “This important discovery of M. Seebeck brings us. . . a step nearer to our object, by referring us to the sun as the great agent of all these phenomena.
” If the copper wires on the globe were replaced by bimetallic strips and heated then “…all the phenomena it now exhibits by aid of the galvanic battery might be represented by the application of heat only.” Finally, Barlow suggested that the differential heating of metals within the earth’s crust was ultimately caused by the sun.
By 1833 Barlow was no longer confident with his earlier ideas. This doubt appeared to spring from difficulties in explaining irregular geomagnetic variations. He now hoped to develop charts of magnetic variation free from theoretical notions. In accordance with this, he left his charts blank in areas where sufficient data were not available. Barlow remarked, “I have offered these few remarks without any intention of their being considered as illustrations of a particular theory… I shall be most happy if our joint labour should furnish the requisite data for. . . [the] development of those mysterious laws which govern the magnetism of the terrestrial globe.”
Barlow’s ideas changed over nearly a twenty-year span. In 1814 he agreed that the earth acted as a giant magnet. From 1819-1822 his experimental results made him increasingly critical of established hypotheses. From 1820 onward he rejected the view which treated the earth as a permanent magnetic body. By 1831 Barlow subsumed all magnetic phenomena to the action of electricity and speculated that the sun’s heat was the ultimate cause of terrestrial magnetism. However, by 1833, he seemingly doubted these notions.
Barlow’s research fits within the context of early nineteenth century geomagnetical studies. His support for the cosmical view was effected by the discoveries Oersted, Ampere, Seebeck, and others. This is evident in his unification of previously disparate forces of nature: magnetism, electricity, heat. As with others supporting the cosmical view, he did not strictly separate geomagnetic measurements in the field and measurements made in laboratory experiments.
This is exemplified in his analogy between the iron ball and the earth. In addition, Barlow’s work illustrates that “pure” and “applied” aspects of terrestrial magnetism were closely linked. Finally, Barlow’s emphasis on careful accumulation of observations in hopes of deriving general laws was typical of the Humboldtian approach to nature.
The Menai Bridge across the Menai Strait
Barlow also worked on the design of bridges, in particular working from 1819 to 1826 with Thomas Telford on the design of the bridge over the Menai Strait. It was the first major modern suspension bridge. Westminster Bridge was built across the Thames in London, despite opposition from city merchants, in the 1740s and rebuilt by Charles Labelye in the 1750s. However when there was a move to demolish and replace this old bridge in the 1820s, Barlow was consulted to compute how the tides on the Thames would be affected by the removal of this structure. After Barlow reported, New London Bridge was designed by John Rennie Sr and built by his son John Rennie Jr.
Barlow was active during the period of railway building in Britain. George Stephenson was the first to build railways in Britain. In 1825 the first train ran from Darlington to Stockton while the 30-mile line from Liverpool and Manchester was opened to traffic in 1830. Stephenson, who constructed both projects, believed that gradients should be less than 1 percent and that curves should have very wide radii of at least a kilometre.
Barlow was appointed as royal commissioner for railways and in the 1830s and 1840s he conducted several experiments to see if the limitation on gradients and radius of curvature proposed by Stephenson was correct. He also tried to determine the most efficient shape for the rails.
In 1822 Barlow built a device which is to be considered one of the first models of an electric motor supplied by continuous current. Mercury is poured into the trough located on the base of the apparatus. The wheel is lowered until a spoke just dips into the mercury. Voltage applied to the binding posts will cause rotation of the wheel.
“The points of the wheel, R, dip into mercury contained in a groove hollowed in the stand. A more rapid revolution will be obtained if a small electro-magnet be substituted for a steel magnet, as is shown in the cut. The electro-magnet is fixed to the stand, and included in the circuit with the spur-wheel, so that the current flows through them in succession. Hence the direction of the rotation will not be changed by reversing that of the current; since the polarity of the electromagnet will also be reversed.” (from the 1842 edition of the Manual of Magnetism, p. 94)
Barlow’s wheel motor
This device, which is to be considered one of the first models of an electric motor supplied by continuous current, was projected and built in 1822 by Peter Barlow. The original model differed from Nobili’s because it had a toothed wheel in the form of a star. Nobili gave the following description of this instrument: “This instrument consists of two parts and of an appendix. The two parts are: first, a small square wooden base, which has two cavities, one in the form of a cup and the other in the form of a container; second, a small brass wheel that can move between the arms of a double square, which bears it above the container. The appendix consists of a small horse shoe-shaped magnet mounted into a small base.
In order to make the small wheel of the mill rotate, one proceeds in the following way. The container is filled with mercury up to the level of the wheel, which the mercury must barely touch. More mercury is poured into the second cavity, where the foot of the double square is fixed. Then the appendix is placed in such a way that the wheel can freely rotate between the arms of the two magnets.
Those arms must pass slightly beyond the point in which the small wheel communicates with mercury. After having placed the device in this way, it is necessary to place the wheel into the voltaic circuit so that one of the connecting wires reaches the cup and the other the container. In the course of this last action the small wheel rotates according to the direction of the current (Barlow)” [Nobili 1834].
He resigned his post at Woolwich Academy in 1847, his public services recognized by the continuance of full pay. Peter Barlow died on the 1st of March 1862, aged 86.